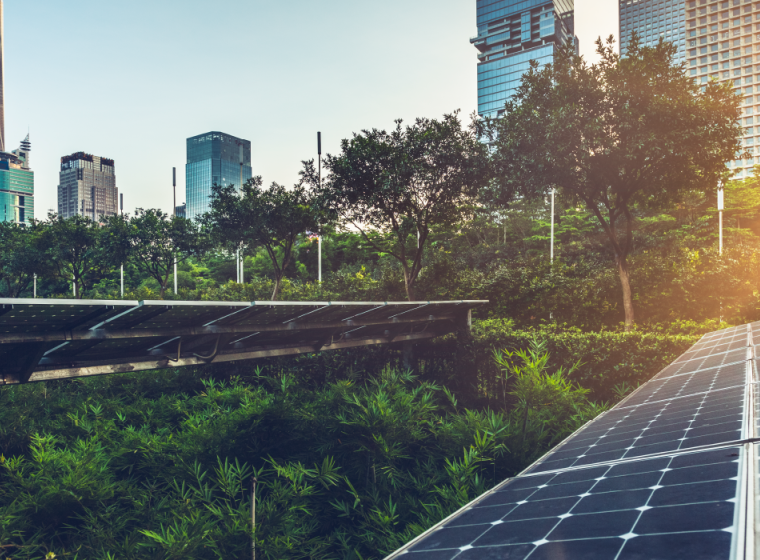
Thought Leadership & Industry Analysis
Facing a complex challenge that requires both industry experience and technical expertise? See how we solve.
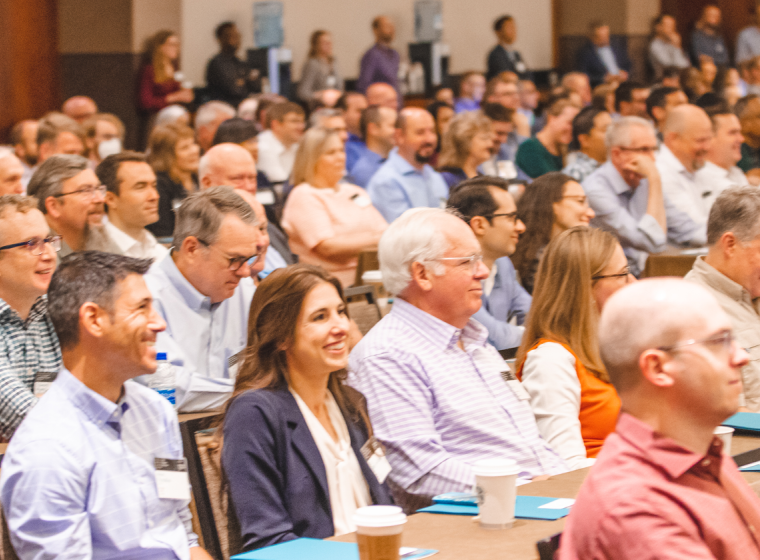
Events & Webinars
Connect with Exponent's multidisciplinary experts at live events and webinars.
![Occupational & Environmental Health Risk Assessment [HS]](/sites/default/files/styles/cards_home_card/public/media/images/GettyImages-949947294.jpg?itok=xAhGlPlV)
Alerts
What you need to know now across more than a dozen industries.
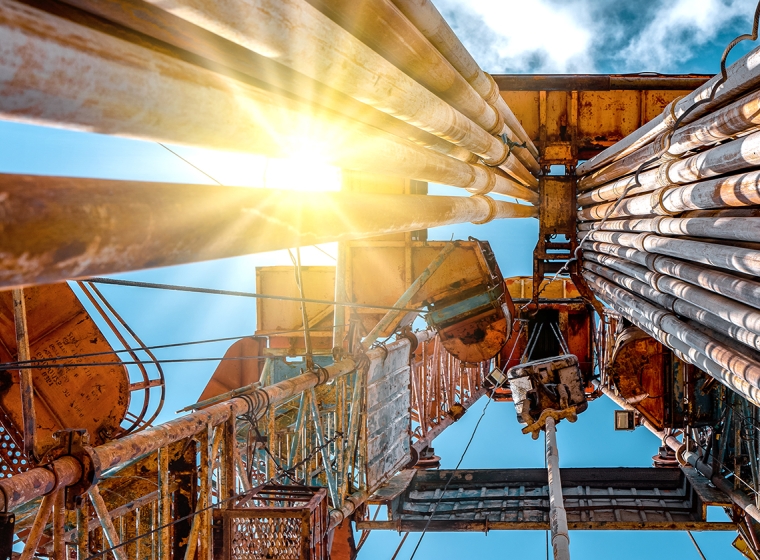
Case Studies
Learn how we apply multidisciplinary thinking to build bespoke solutions for clients in the real world.

Publications
When we're not dedicated to clients, we're advancing engineering and scientific research in 90+ disciplines.
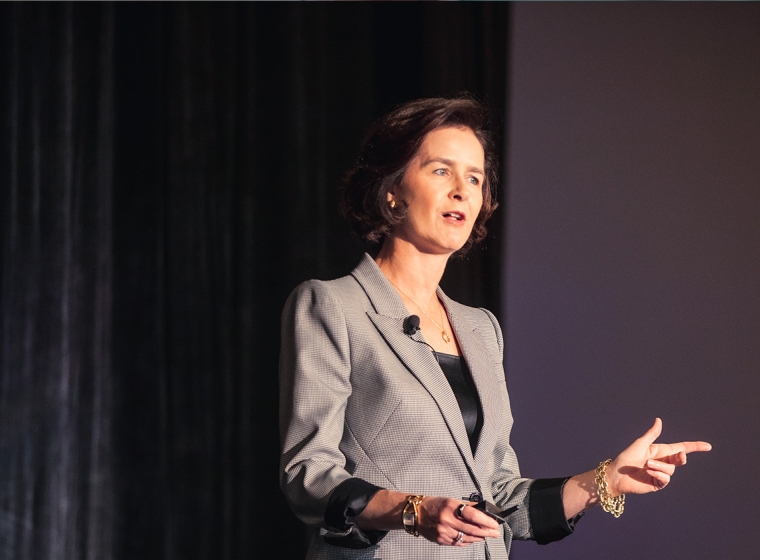
Announcements
Read about Exponent's people, partnerships, and other key firm news.