September 15, 2022
Using network-level risk assessment to prioritize infrastructure interventions
Disruptions to infrastructure networks can result in cascading impacts that, in the worst cases, endanger the communities those networks should serve. Millions of Texans endured weeks of below-freezing temperatures as well as food and water shortages when the state's electric grid failed in February 2021. In California, intentional power outages designed to reduce the risk of wildfires also affect people with disabilities and other vulnerable populations.
Critical infrastructure networks in the U.S. are known to be vulnerable to disruptions. On their 2021 Report Card for America's Infrastructure, the American Society of Civil Engineers graded the nation's many infrastructure systems at a C- overall. Transit systems score a D-, roads a D, both energy and drinking water systems a C-, and bridges a C.
Last year's infrastructure legislation provides $550 billion for new investments in transportation, water, and energy networks, as well as in other systems and their resilience. Decision-makers tasked with allocating these funds — already eroded by inflation — now face a stiff challenge. With infrastructure in poor repair, subject to multiple, evolving hazards, and expected to serve communities that will continue to grow and change over the service lives of their components, how should interventions in these networks be prioritized?
Critical Infrastructure
Component- vs. network-level risk assessment
The risk of a component failing is the product of its probability of failure and the consequences of that failure. Quantitative risk assessment methods that estimate the risk of failure for single components in a network can help to first identify and then strengthen weak points within that system. However, such methods do not capture how component failures affect the performance of the system as a whole. That is, what is the consequence of a component failure for the people or other networks that rely on the system to provide a minimum level of service?
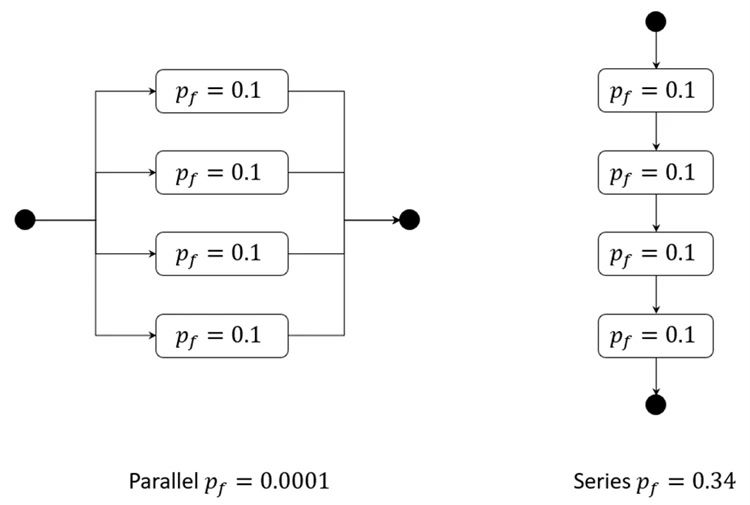
Figure 1. The network failure probability of four components in parallel compared to the same four components in series.
Consider the simple example in Figure 1. Even though the failure probability of each individual component is the same, the way the components are connected leads to vastly different network failure probabilities. The parallel system is significantly more robust than the series system. However, a component-level risk assessment would not make any distinction between the series system and the parallel system and would therefore be misleading.
While it is often more computationally expensive to model network performance than to focus on individual components, there are many reasons doing so may be worth the additional effort. Evaluating system performance is a prerequisite for assessing community impacts from network disruptions. Network modeling enables analysts to better understand the impact of component-level interventions on overall performance and resilience, and it enables decision-makers to understand who is affected by network disruptions.
Example: changing traffic patterns due to aging bridges
Aging bridges may become "structurally deficient," a designation that typically triggers more frequent structural inspections and reductions in those bridges' load ratings. Lower load ratings reduce traffic capacity, so a four-lane bridge might have only two lanes open, or it may no longer be able to service trucking routes that are critical to supply chains or buses that convey many passengers simultaneously and reduce congestion.
In turn, the larger traffic networks that rely on those bridges may see more congestion as drivers adjust their routes, with freight vehicles opting for appropriately rated routes, commuters seeking to avoid truck traffic, and other drivers trying to bypass both. Conversely, some drivers may opt to use public transit, decongesting roads and reducing demand on bridges but increasing demand on bus, subway, and light-rail systems.
A component-level risk assessment might quantify the probability of individual bridges becoming structurally deficient in the next decade. A network-level risk assessment could go a step further, helping to answer questions including:
- How do changes in bridges' load ratings affect drivers' average travel times?
- How might the risk of travel delays evolve over time as bridges age and communities grow and change?
- How might regional demand for public transit change as a result of bridges aging? Where would future investments in public transit most effectively meet that potential demand?
- Which communities' trips are most and least impacted by structurally deficient bridges?
- How are impacts on drivers' well-being distributed among different demographic groups and geographic areas?
Answering these and similar questions could help road network managers develop more equitable mitigation strategies that account for the impacts of aging bridges on different groups of drivers.
Example: unscheduled power outages due to contamination-induced insulator flashover
Insulators connect conductors to supporting structures, like towers and poles, while preventing the flow of current out of the conductors. Over its decades-long service life, an insulator will be exposed to airborne contaminants that may accumulate on its surface. Flashover can occur when heavy fog or very light rain comes into contact with salts in the contamination on the insulator's surface, creating a conductive pathway for the current carried by the power line to follow (Figure 2). Consequences range from insulator damage to power outages or wildfires.

Figure 2. Events that can lead to contamination-induced insulator flashover.
An electric utility's service area may include millions of transmission and distribution structures and even more insulators. A component-level risk assessment could provide valuable insight into regions or circuits where insulators have a greater risk of contamination-induced flashover. Those regions may still be large, however, and decision-makers may wish to further prioritize insulators within them.
Modeling the performance of the electric grid could enable decision-makers to develop prioritization strategies informed by resilience and equity considerations. Questions that a network-performance-based risk assessment approach could help answer include:
- How do different possible manual washing programs impact the resilience of the electric grid?
- Which communities are most at risk for unscheduled power outages resulting from contamination-induced insulator flashover?
- How does the risk of unscheduled power outages vary across demographic groups within the region of interest?
- How might we ensure that we are protecting people for whom power outages are life-threatening events?
- Which communities have the most limited capacity to adapt to unplanned power outages and therefore should receive more attention in risk-reduction measures?
- How might the risks associated with blackouts due to contamination-induced insulator flashover evolve with climate change and increasing demand for electric power?
Understanding how the network serves the people who rely on it — and who can be made vulnerable by system failures — allows decision-makers to interrogate how community impacts are distributed among different demographics. Network-level probabilistic risk assessment can help ensure the potential impacts associated with network disruptions are not borne by the most vulnerable groups of people.
How Exponent Can Help
Exponent's Buildings & Structures practice specializes in developing quantitative risk assessment strategies for a variety of systems and hazards in partnership with in-house domain experts for utilities including electric power transmission and distribution grids, oil and gas pipelines, water and wastewater systems, and road networks. By leveraging the expertise of our Data Sciences practice, we can implement computationally efficient models to simulate the performance of large infrastructure networks.
We can help to:
- Develop probabilistic risk assessment methods for infrastructure networks subject to multiple, uncertain, or evolving hazards.
- Assess and disaggregate the community impacts of network disruptions.
- Optimize component repair and upgrade schedules.
- Inform inspection plans.
- Identify the components that play the biggest role in the performance of a network subject to an uncertain hazard.
Insights
