How does Exponent's multidisciplinary approach provide cutting-edge insights for battery technologies?
From implantable medical devices to grid-scale storage systems, electric cars, and interconnected smart devices — our clients build the battery-enabled products that power daily life and are poised to play an even bigger role in the future.
At Exponent, we believe making that future a reality requires understanding how batteries have evolved, as well as the many ways they may fail. The battery technology space is increasingly competitive, and our clients require trusted, objective guidance and innovative battery performance evaluation and testing capabilities that enable them to bring products to market with confidence.
Leveraging our deep history in failure analysis and unparalleled multidisciplinary expertise, Exponent's energy storage and battery technology consultants bring a unique focus to helping ensure performance, reliability, and safety at every stage of the product lifecycle.
Whether addressing a design component issue, conducting a failure analysis investigation, sourcing global manufacturing, tracing quality concerns across complex supply chains, or prioritizing corrective actions, Exponent works with batteries of every size and scale. Our experts can step in at any point to address specific issues or serve as a partner of choice for the full product journey. Our work encompasses a broad range of industries, including medical devices, consumer products and electronics, automated and electric mobility, and grid-scale utilities/energy storage.
Capabilities
Through our range of capabilities, rigorous testing methods, and state-of-the-art facilities, we help clients understand the performance, safety, and reliability of their products throughout their lifecycle.
Experts
Our global and comprehensive expertise across industries gives us a deep understanding of current challenges, best industry practices, and the implications of emerging technologies.
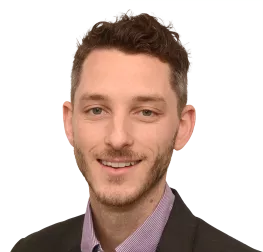
Corporate Vice President and Principal Engineer
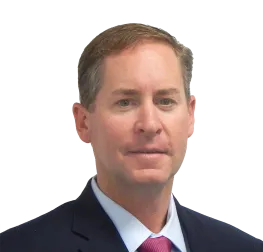
Corporate Vice President, Office Director and Principal Engineer
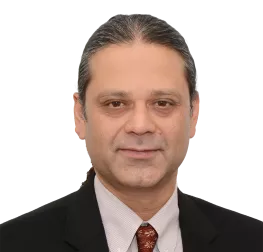
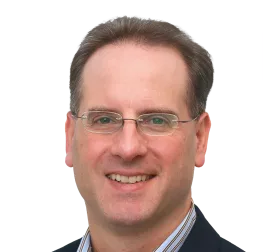

Office Director and Principal Engineer
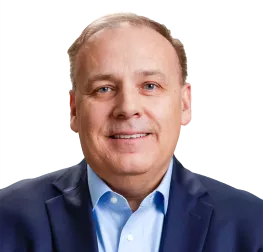
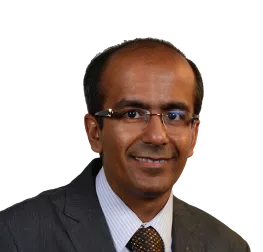
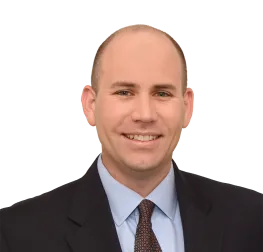
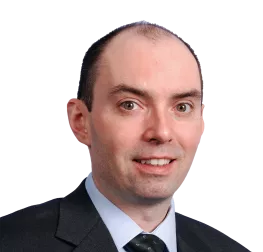
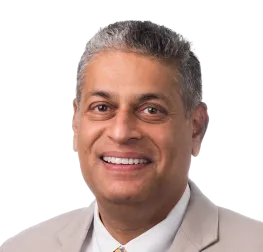
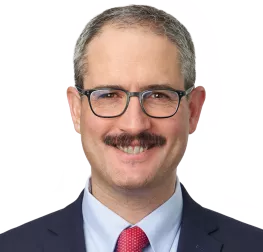
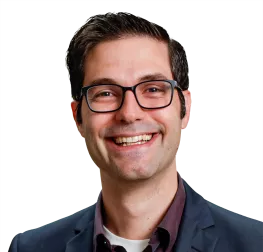
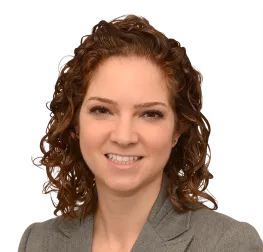
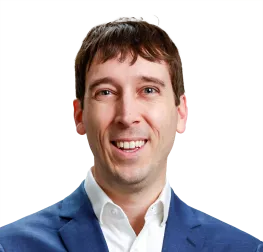
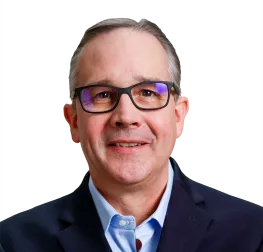
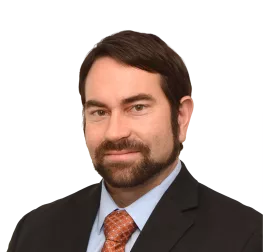
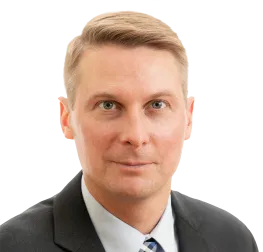
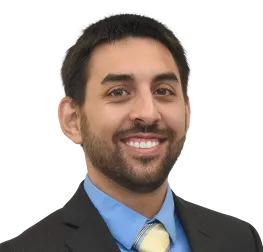
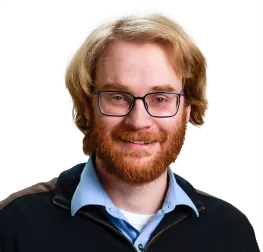
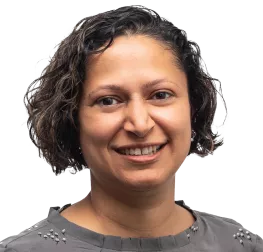
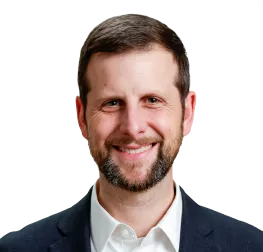
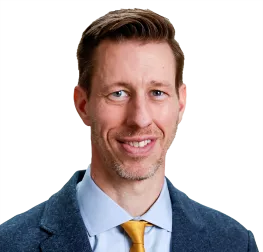
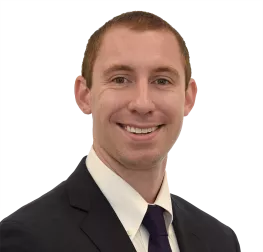
Senior Managing Engineer
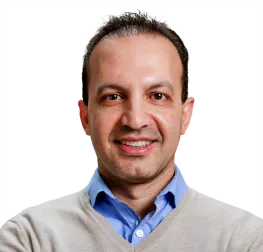
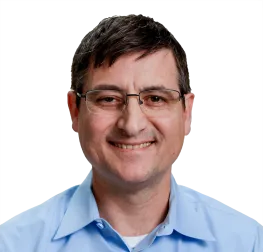
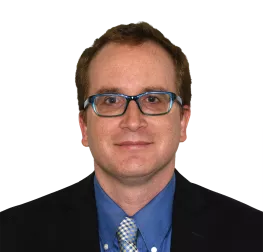
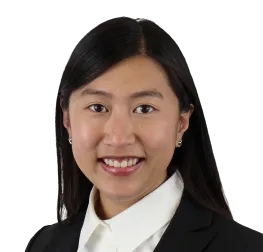
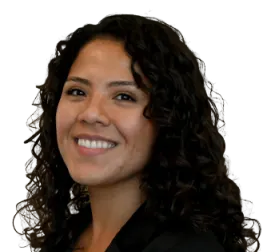
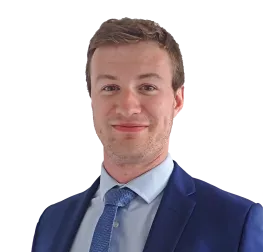
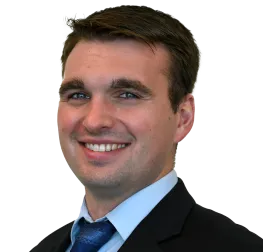
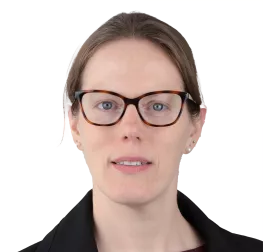
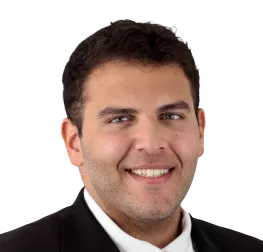
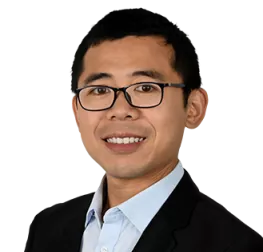
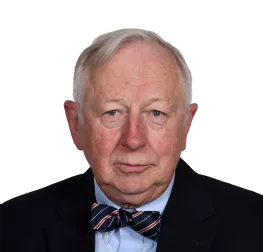
Our Capabilities Are Unparalleled
Our battery and energy storage experts can step in at any point to address specific issues or serve as a partner of choice for the battery product journey. Our work encompasses a broad range of industries, including medical devices, consumer products and electronics, automated and electric mobility, and grid-scale utilities/energy storage.

Batteries & Energy Storage
Supercharge performance, reliability, and safety across all stages of the battery and energy storage product lifecycle.
![[MCE] Factory Auditing & Assembly Line Evaluations - view of assembly line floor](/sites/default/files/styles/cards_home_card/public/media/images/GettyImages-1320950393.jpg.webp?itok=JflDDR6c)
Materials & Corrosion Industry Expertise
Materials science engineering expertise tailored for every industry.
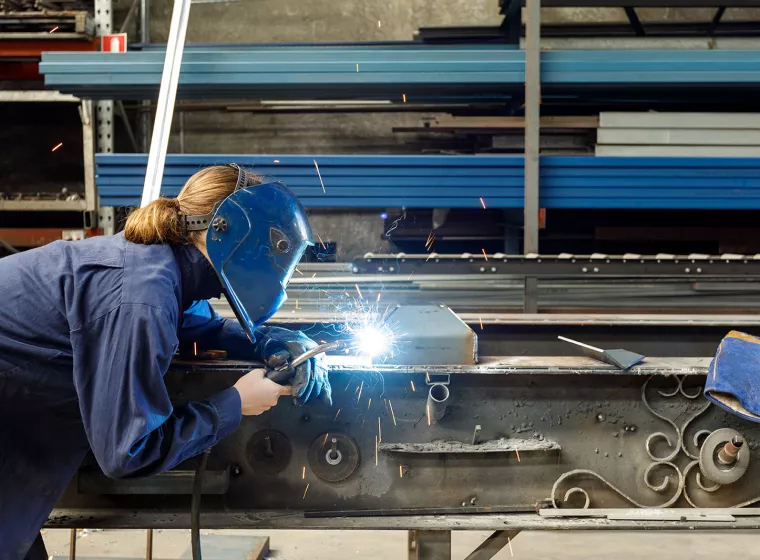
Product & Process Advisory
Materials and corrosion engineers assist with materials issues at every stage of your product or process lifecycle.

Materials, Metallurgy & Corrosion Engineering Capabilities
Assess corrosion risks and follow best practices in materials selection, operations, and corrosion control for metals.
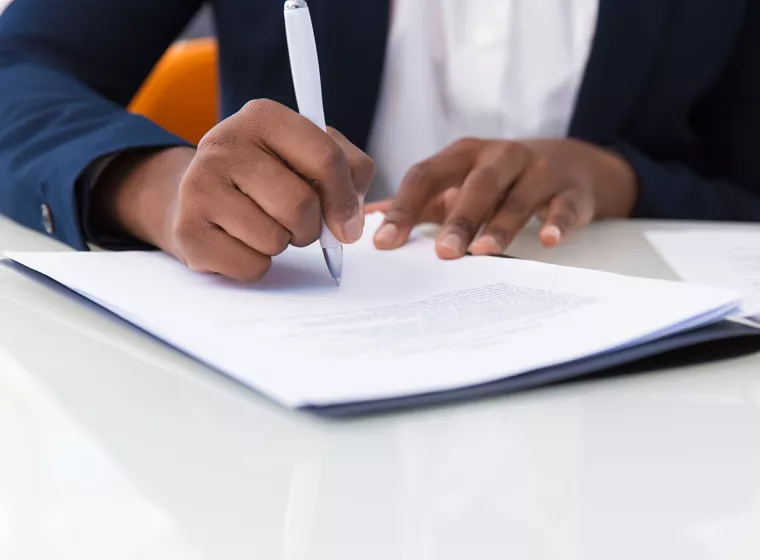
Materials & Corrosion Engineering Expertise for Disputes
Technical scientific support for disputes involving materials and corrosion.